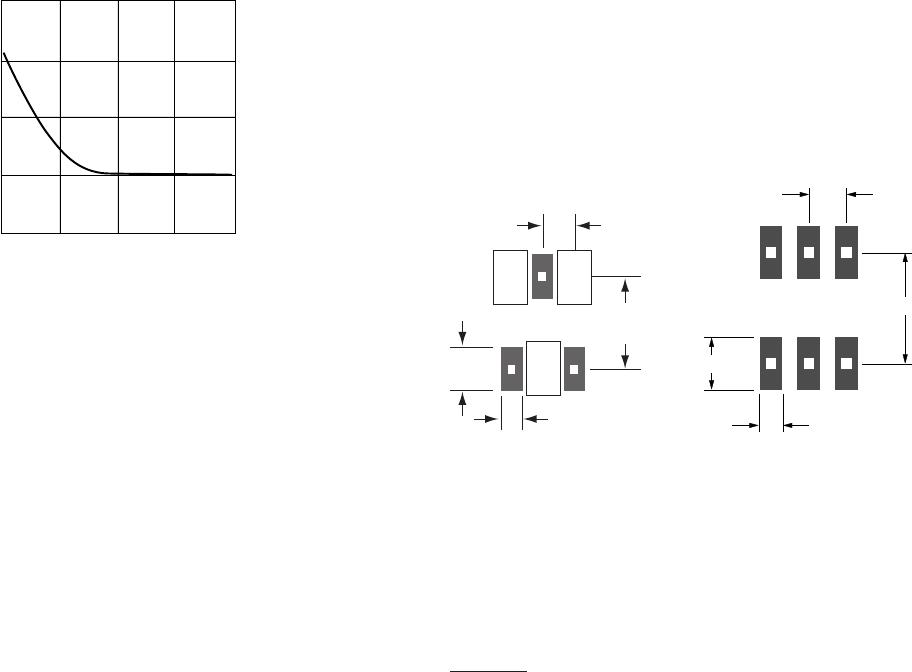
8
Such a circuit oers several advantages. First the voltage
outputs of two diodes are added in series, increasing the
overall value of voltage sensitivity for the network (com-
pared to a single diode detector). Second, the RF imped-
ances of the two diodes are added in parallel, making
the job of reactive matching a bit easier. Such a circuit
can easily be realized using the two series diodes in the
HSMS-285C.
Flicker Noise
Reference to Figure 5 will show that there is a junc-
tion of metal, silicon, and passivation around the rim
of the Schottky contact. It is in this three-way junction
that icker noise
[5]
is generated. This noise can severely
reduce the sensitivity of a crystal video receiver utiliz-
ing a Schottky detector circuit if the video frequency is
below the noise corner. Flicker noise can be substantially
reduced by the elimination of passivation, but such
diodes cannot be mounted in non-hermetic packages.
p-type silicon Schottky diodes have the least icker noise
at a given value of external bias (compared to n-type
silicon or GaAs). At zero bias, such diodes can have
extremely low values of icker noise. For the HSMS-285x
series, the noise temperature ratio is given in Figure 14.
Any Schottky junction, be it an RF diode or the gate of
a MESFET, is relatively delicate and can be burned out
with excessive RF power. Many crystal video receivers
used in RFID (tag) applications nd themselves in poorly
controlled environments where high power sources may
be present. Examples are the areas around airport and
FAA radars, nearby ham radio operators, the vicinity of
a broadcast band transmitter, etc. In such environments,
the Schottky diodes of the receiver can be protected
by a device known as a limiter diode.
[6]
Formerly avail-
able only in radar warning receivers and other high cost
electronic warfare applications, these diodes have been
adapted to commercial and consumer circuits.
Avago oers a complete line of surface mountable
PIN limiter diodes. Most notably, our HSMP-4820 (SOT-
23) can act as a very fast (nanosecond) power-sensi-
tive switch when placed between the antenna and the
Schottky diode, shorting out the RF circuit temporar-
ily and reecting the excessive RF energy back out the
antenna.
Assembly Instructions
SOT-323 PCB Footprint
A recommended PCB pad layout for the miniature SOT-
323 (SC-70) package is shown in Figure 15 (dimensions
are in inches). This layout provides ample allowance for
package placement by automated assembly equipment
without adding parasitics that could impair the perfor-
mance. Figure 16 shows the pad layout for the six-lead
SOT-363.
[4]
Avago Application Note 956-4, Schottky Diode Voltage Doubler.
[5]
Avago Application Note 965-3, Flicker Noise in Schottky Diodes.
[6]
Avago Application Note 1050, Low Cost, Surface Mount Power Limiters.
NOISE TEMPERATURE RATIO (dB)
FREQUENCY (Hz)
15
10
5
0
-5
10 100 1000 10000 100000
Diode Burnout
Figure 14. Typical Noise Temperature Ratio.
Noise temperature ratio is the quotient of the diode’s
noise power (expressed in dBV/Hz) divided by the noise
power of an ideal resistor of resistance R = R
V
.
For an ideal resistor R, at 300°K, the noise voltage can be
computed from
v = 1.287 X 10
-10
√R volts/Hz
which can be expressed as
20 log
10
v dBV/Hz
Thus, for a diode with R
V
= 9 KΩ, the noise voltage is
12.2 nV/Hz or -158 dBV/Hz. On the graph of Figure 14, -
158 dBV/Hz would replace the zero on the vertical scale
to convert the chart to one of absolute noise voltage vs.
frequency.
0.026
0.039
0.079
0.022
Dimensions in inches
Figure 15. Recommended PCB
Pad Layout for Avago’s SC70
3L/SOT-323 Products.
Figure 16. Recommended PCB Pad
Layout for Avago's SC70 6L/SOT-363
Products.