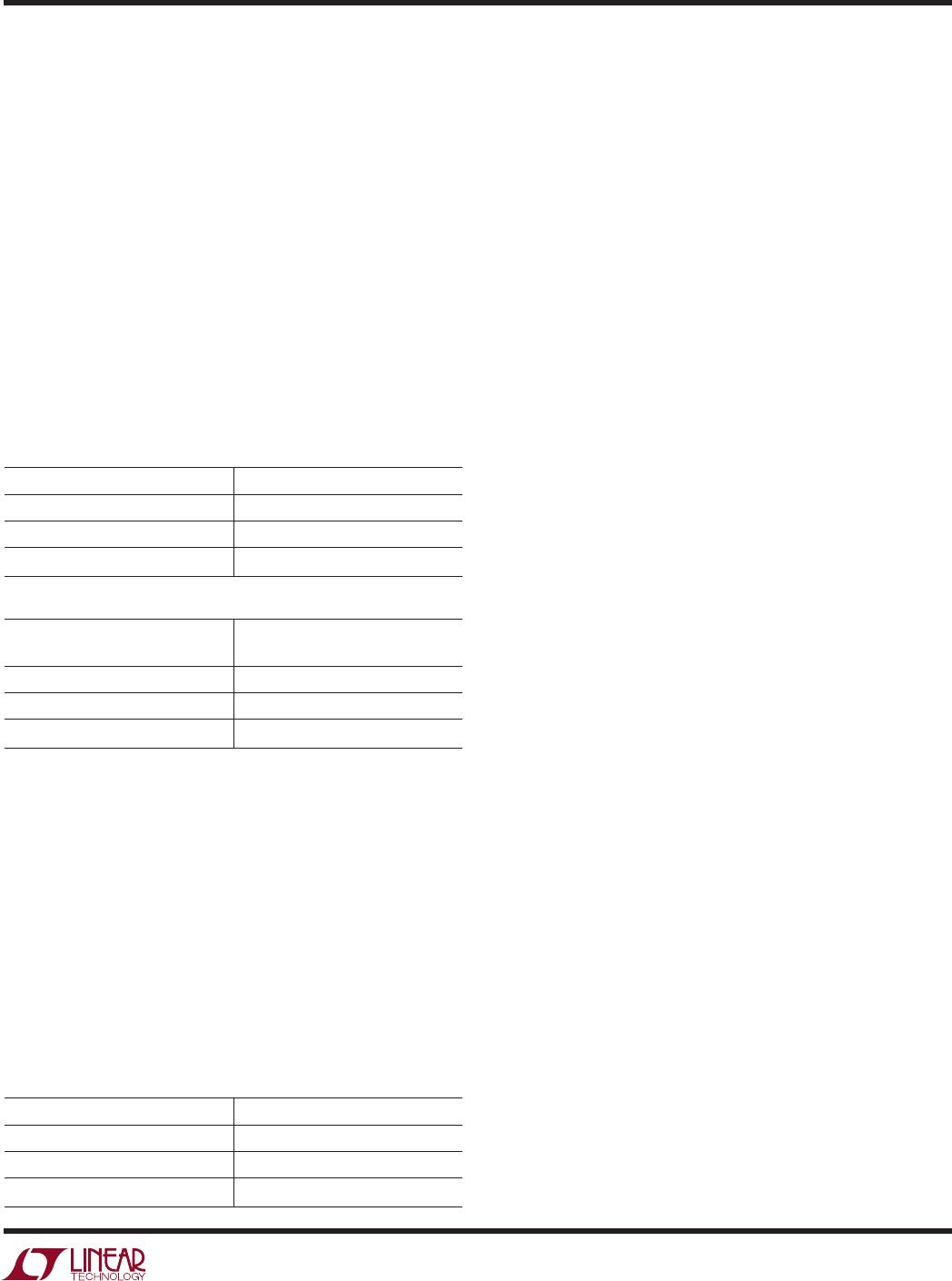
9
LTC1569-6
DC Accuracy
DC accuracy is defined as the error in the output voltage
after DC offset and DC gain errors are removed. This is
similar to the definition of the integral nonlinearity in A/D
converters. For example, after measuring values of V
OUT(DC)
vs V
IN(DC)
for a typical LTC1569-6, a linear regression
shows that V
OUT(DC)
= V
IN(DC)
• 0.99854 + 0.00134V is the
straight line that best fits the data. The DC accuracy
describes how much the actual data deviates from this
straight line (i.e., DCERROR = V
OUT(DC)
– (V
IN(DC)
• 0.99854
+ 0.00134V). In a 12-bit system with a full-scale value of
2V, the LSB is 488µV. Therefore, if the DCERROR of the
filter is less than 488µV over a 2V range, the filter has
12-bit DC accuracy. Figure 9 illustrates the typical DC
accuracy of the LTC1569-6 on a single 5V supply.
DC Offset
The output DC offset of the LTC1569-6 is trimmed to less
than ±5mV. The trimming is performed with V
S
= 1.9V,
–1.1V with the filter cutoff frequency set to 4kHz (R
EXT
=
10k, DIV/CLK shorted to V
+
). To obtain optimum DC offset
performance, appropriate PC layout techniques should be
used. The filter IC should be soldered to the PC board. The
power supplies should be well decoupled including a 1µF
ceramic capacitor from V
+
(Pin 7) to V
–
(Pin 4). A ground
plane should be used. Noisy signals should be isolated
from the filter input pins.
When the power supply is 3V, the output DC offset should
change less than ±2mV when the clock frequency varies
from 64kHz to 4096kHz. When the clock frequency is
fixed, the output DC offset will typically change by less
than ±3mV (±15mV) when the power supply varies from
3V to 5V (±5V) in the divide-by-1 mode. In the divide-by-
4 or divide-by-16 modes, the output DC offset will typically
change less than –9mV (–27mV) when the power supply
varies from 3V to 5V (±5V). The offset is measured with
respect to GND (Pin 3).
Aliasing
Aliasing is an inherent phenomenon of sampled data
filters. In lowpass filters significant aliasing only occurs
when the frequency of the input signal approaches the
sampling frequency or multiples of the sampling fre-
APPLICATIONS INFORMATION
WUU
U
f
CUTOFF
), as shown in the Typical Applications section. The
typical variation in dynamic input impedance for a given
clock frequency is ±10%.
Wideband Noise
The wideband noise of the filter is the RMS value of the
device’s output noise spectral density. The wideband
noise data is used to determine the operating signal-to-
noise at a given distortion level. The wideband noise is
nearly independent of the value of the clock frequency and
excludes the clock feedthrough. Most of the wideband
noise is concentrated in the filter passband and cannot be
removed with post filtering (Table 2). Table 3 lists the
typical wideband noise for each supply.
Table 2. Wideband Noise vs Supply Voltage, Single 3V Supply
Bandwidth Total Integrated Noise
DC to f
CUTOFF
80µV
RMS
DC to 2 • f
CUTOFF
95µV
RMS
DC to f
CLK
110µV
RMS
Table 3. Wideband Noise vs Supply Voltage, f
CUTOFF
= 64kHz
Total Integrated Noise
Power Supply DC to 2 • f
CUTOFF
3V 95µV
RMS
5V 100µV
RMS
±5V 105µV
RMS
Clock Feedthrough
Clock feedthrough is defined as the RMS value of the clock
frequency and its harmonics that are present at the filter’s
OUT pin (Pin 8). The clock feedthrough is measured with
IN
+
and IN
–
(Pins 1 and 2) grounded and depends on the
PC board layout and the power supply decoupling. Table␣ 4
shows the clock feedthrough (the RMS sum of the first 11
harmonics) when the LTC1569-6 is self-clocked with
R
EXT
= 10k, DIV/CLK (Pin 5) open (divide-by-4 mode). The
clock feedthrough can be reduced with a simple RC post
filter.
Table 4. Clock Feedthrough
Power Supply Feedthrough
3V 0.1mV
RMS
5V 0.3mV
RMS
±5V 0.9mV
RMS