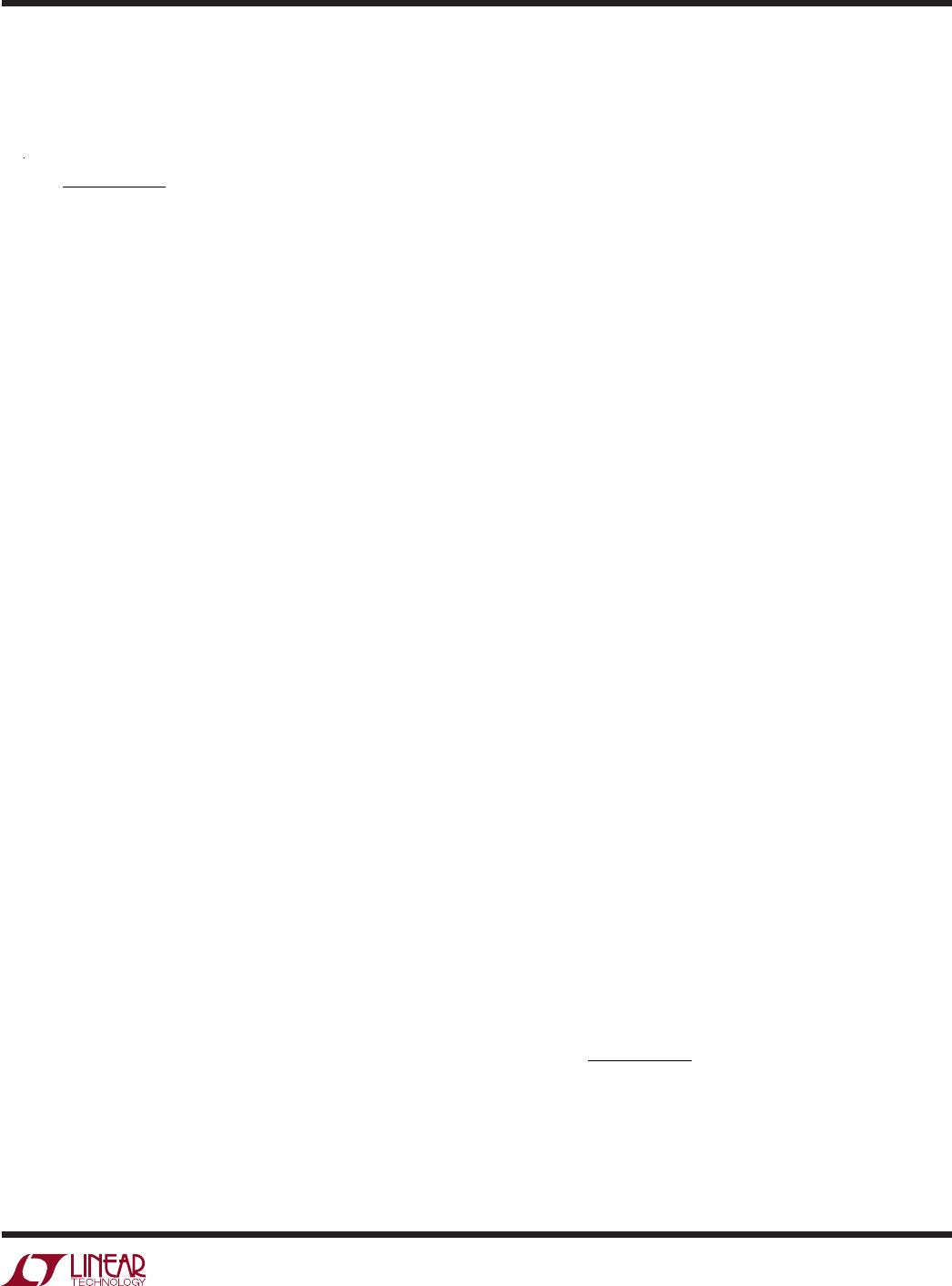
LTC3626
19
3626fa
For more information www.linear.com/LTC3626
applicaTions inForMaTion
series with the compensation capacitor. The resistor in
series with the capacitor creates a zero in the current limit
loop transfer function given by:
f
Z
=
2 • π •R
•
while minimally impacting the frequency of the compensa-
tion pole. Given the current limit loop frequency response
contains several moderate frequency poles: one at approxi-
mately
10
kHz (typical) and two at approximately 100kHz
(typical),
the placement of the zero in frequency can be
used to provide additional phase margin, which in turn,
may allow a higher loop bandwidth without sacrificing
loop stability. For example, choosing C = 0.33µF and R
Z
=
50 creates a zero at approximately 10kHz thereby reduc-
ing the impact of the internal pole located at that same
frequency
.
With this compensation scheme, the LTC3626
current limit loop will have a dominant pole frequency, and
overall loop bandwidth, roughly three times higher than
that provided with a 1µF capacitor, while likely providing
adequate loop stability.
As previously described, the LTC3626 senses the average
output current through the synchronous FET during the
off time. As a result, it is recommended the LTC3626 be
operated with an off time of greater than 150ns for best
current monitor accuracy. For many applications, this
is of little concern unless operating at or near regulator
dropout conditions (extremely high duty-cycle operation)
and high switching frequencies. Overall, best current
monitor accuracy is achieved with output currents above
approximately 200mA in forced continuous mode with
switching frequencies of 1MHz or lower.
On-Die Temperature Monitor and Limit
The LTC3626 produces a voltage at the TMON pin propor
-
tional to the measured junction temperature. The junction
temperature-to-voltage scaling factor is 200°K/V.
Thus,
to obtain the junction temperature in degrees Kelvin,
simply multiply the voltage provided at the TMON pin by
the scaling factor. To obtain the junction temperature in
degrees Celsius, subtract 273 from the value obtained in
degrees Kelvin.
The temperature monitor function uses a chopping tech
-
nique to achieve high precision. As a result
, a small periodic
ripple may be seen at the TMON pin, the average of which
is the measured value of interest. The ripple frequency will
be the operating frequency divided by 32. If required, a
1µF or greater capacitor to SGND may be placed on the
output to reduce the magnitude of the ripple.
The temperature monitor output is driven from a flexible,
internally compensated on-chip buffer capable of sourcing
or sinking small amounts of continuous currents (<20µA
typical). The buffer internal compensation is intended
for capacitive loads up to approximately 150pF (typical).
This configuration allows direct connection of TMON to
convenient test equipment, such as a multimeter, for
temperature measurement. The internal compensation
may be overridden by connecting a capacitor of value 1µF
or greater between TMON and SGND. This configuration
allows for a wide range of applications requiring stability
with higher load capacitance, such as some ADC inputs.
The voltage produced at TMON is continuously fed to
a limit comparator that has the voltage at the TSET pin
as its reference input. When triggered, this comparator
generates an overtemperature fault that will initiate part
shutdown and reset of soft-start. Thus, a programmable
temperature limit may be obtained by providing a voltage
at the TSET pin that corresponds to the temperature limit
of interest. The voltage at the TSET pin may be derived
from a resistor divider from INTV
CC
, subject to the current
constraints listed in the Electrical Characteristics section,
or may be driven externally. The LTC3626 will clear the
overtemperature fault and attempt to restart once the in
-
ternal temperature falls 10°C (typical)
from the threshold
given at TSET. As an example, to set a temperature limit
at approximately 125°C, the voltage at TSET should be:
V
TSET
=
°
+
200
K/V
≈ 2V