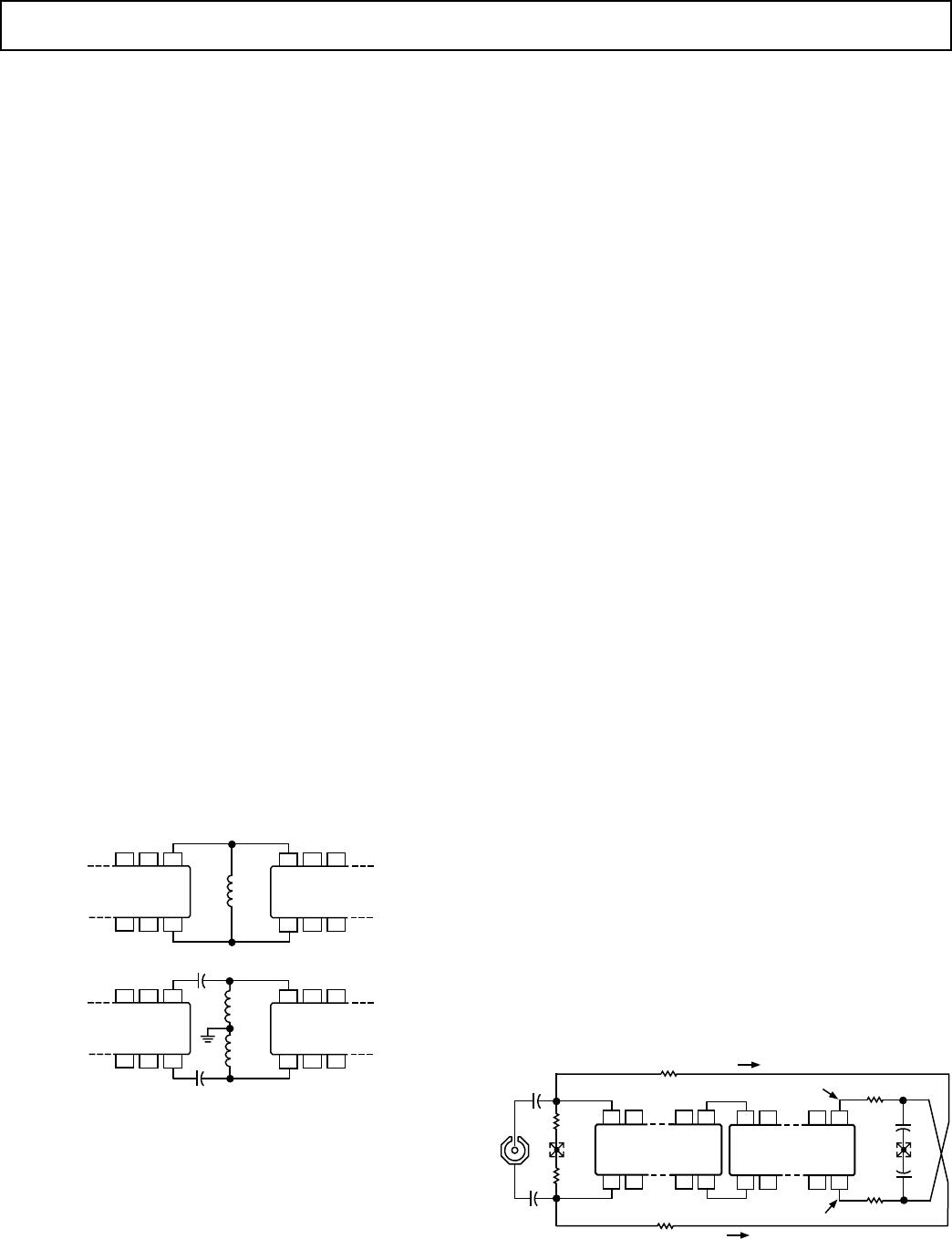
REV. D
AD641
–13–
OPERATION OF CASCADED AD641S
Frequently, the dynamic range of the input will be 50 dB or
more. Two AD641s can be cascaded, as shown in Figure 27.
The balanced signal output from U1 becomes the input to U2.
Resistors are included in series with each LOG OUT pin and
capacitors C1 and C2 are placed directly between Pins 13 and 14
to provide a local path for the RF current at these output pairs.
C1 through C3 are chosen to provide the required low pass
corner in conjunction with the load R
L
. Board layout and
grounding disciplines are critically important at the high gain
(X100,000) and bandwidth (~ 150 MHz) of this system.
The intercept voltage is calculated as follows. First, note that if
its LOG OUT is disconnected, U1 simply inserts 50 dB of gain
ahead of U2. This would lower the intercept by 50 dB, to
–110 dBV for square wave calibration. With the LOG OUT of
U1 added in, there is a finite zero signal current which slightly
shifts the intercept. With the intercept temperature compensa-
tion on U1 disabled this zero signal output is –270 µA equiva-
lent to a 5.4 dB upward shift in the intercept, since the slope is
50 µA/dB. Thus, the intercept is at –104.6 dBV (–88 dBm for
50 Ω sine calibration). ITC may be disabled by grounding Pin 8
of either U1 or U2.
Cascaded AD641s can be used in dc applications, but input
offset voltage will limit the dynamic range. The dc intercept is
6 µV. The offset should not be confused with the intercept, which is
found by extrapolating the transfer function from its central “log
linear” region. This can be understood by referring to Equation
(1) and noting that an input offset is simply additive to the value
of V
IN
in the numerator of the logarithmic argument; it does not
affect the denominator (or intercept) V
X
. In dc coupled applica-
tions of wide dynamic range, special precautions must be taken
to null the input offset and minimize drift due to input bias
offset. It is recommended that the input attenuator be used,
providing a practical input range of –74 dBV (±200 µV dc) to
+6 dBV (±2 V dc) when nulled using the adjustment circuit
shown in Figure 25.
1920
21
U2
12
11
910
U1
1920
21
U2
12
11
910
U1
(a)
b
Figure 28. Two Methods for AC Coupling AD641s
Eliminating the Effect of First Stage Offset
Usually, the input signal will be sinusoidal and U1 and U2 can
be ac coupled. Figure 28a shows a low resistance choke at the
input of U2 which shorts the dc output of U1 while preserving
the hf response. Coupling capacitors may be inserted (Figure
28b) in which case two chokes are used to provide bias paths for
U2. These chokes must exhibit high impedance over the operat-
ing frequency range.
Alternatively, the input offset can be nulled by a negative feed-
back network from the SIG OUT nodes of U2 to the SIG IN
nodes of U1, as shown in Figure 29. The low pass response of
the feedback path transforms to a closed-loop high pass response.
The high gain (×100,000) of the signal path results in a com-
mensurate reduction in the effective time constant of this net-
work. For example, to achieve a high pass corner of 100 kHz,
the low pass corner must be at 1 Hz.
In fact, it is somewhat more complicated than this. When the ac
input sufficiently exceeds that of the offset, the feedback be-
comes ineffective and the response becomes essentially dc
coupled. Even for quite modest inputs the last stage will be
limiting and the output (Pins 10 and 11) of U2 will be a square
wave of about ±180 mV amplitude, dwelling approximately
equal times at its two limit values, and thus having a net average
value near zero. Only when the input is very small does the high
pass behavior of this nulling loop become apparent. Consequently,
the low pass time constant can usually be reduced considerably
without serious performance degradation.
The resistor values are chosen such that the dc feedback is
adequate to null the worst case input offset, say, 500 µV. There
must be some resistance at Pins 1 and 20 across which the offset
compensation voltage is developed. The values shown in the
figure assume that we wish to terminate a 50 Ω source at Pin 20.
The 50 Ω resistor at Pin 1 is essential, both to minimize offsets
due to bias current mismatch and because the outputs at Pins
10 and 11 can only swing negatively (from ground to –180 mV)
whereas we need to cater for input offsets of either polarity.
For a sine input of 1 µV amplitude (–120 dBV) and in the ab-
sence of offset, the differential voltage at Pins 10 and 11 of U2
would be almost sinusoidal but 100,000 times larger, or 100 mV.
The last limiter in U2 would be entering saturation. A 1 µV
input offset added to this signal would put the last limiter well
into saturation, and its output would then have a different aver-
age value, which is extracted by the low pass network and deliv-
ered back to the input. For larger signals, the output approaches a
square wave for zero input offset and becomes rectangular when
offset is present. The duty cycle modulation of this output now
produces the nonzero average value. Assume a maximum re-
quired differential output of 100 mV (after averaging in C1 and
C2) as shown in Figure 29. R3 through R6 can now be chosen
to provide ±500 µV of correction range, and with these values
the input offset is reduced by a factor of 500. Using 4.7 µF
capacitors, the time constant of the network is about 1.2 ms,
and its corner frequency is at 13.5 Hz. The closed loop high
pass corner (for small signals) is, therefore, at 1.35 MHz.
20
110
11
U1
A
VE
= –140mV
INPUT
20
110
11
U2
R1
50V
R2
50V
C1
C2
A
VE
= –140mV
R3
4.99kV
R5
4.99kV
–200mV
–700mV
4mA
14mA
R4
4.99kV
R6
4.99kV
Figure 29. Feedback Offset Correction Network