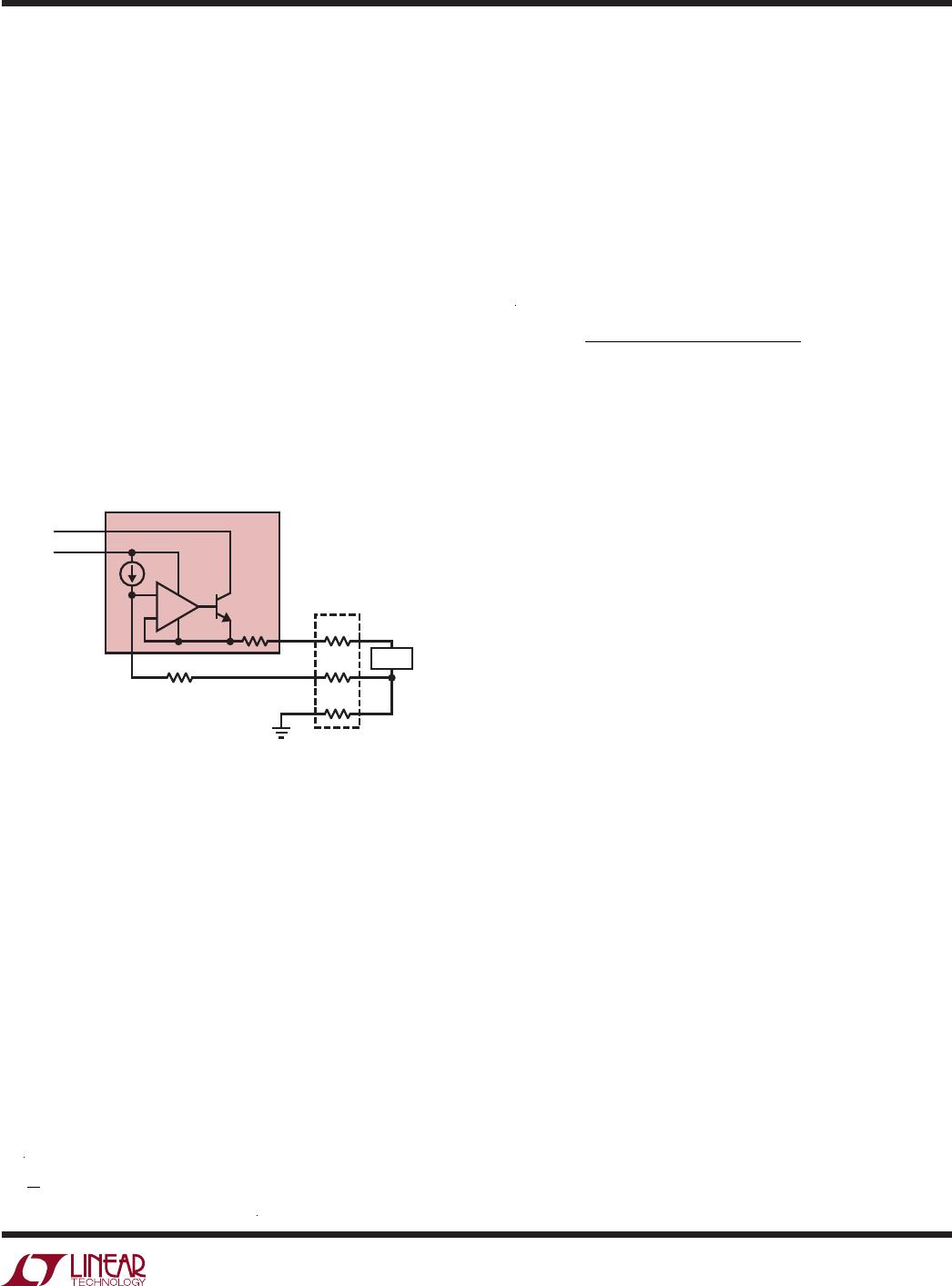
LT3080-1
13
30801fc
For more information www.linear.com/LT3080-1
As output current decreases below the midpoint, output
voltage increases above the nominal set-point. Correspond-
ingly, as output current increases above the midpoint,
output voltage decreases below the nominal set-point
.
During a large output load transient
, output voltage
perturbation is contained within a window that is tighter
than what would result if active voltage positioning is not
employed. Choose the SET pin resistor value by using the
formula below:
R
SET
=
OUT
+
MID
BALLAST
I
where
I
MID
= 1/2 (I
OUT(MIN)
+ I
OUT(MAX)
)
R
BALLAST
= 25mΩ
I
SET
= 10µA
Thermal Considerations
The LT3080-1 has internal power and thermal limiting
circuitry designed to protect it under overload conditions.
For continuous normal load conditions, maximum junc
-
tion temperature must not be exceeded. It is important
to give consideration to all sour
ces of thermal resistance
from junction to ambient
. This includes junction-to-case,
case-to-heat sink interface, heat sink resistance or circuit
board-to-ambient as the application dictates. Additional
heat sources nearby must also be considered.
For surface mount devices, heat sinking is accomplished
by using the heat spreading capabilities of the PC board
and its copper traces. Surface mount heat sinks and plated
through-holes can also be used to spread the heat gener
-
ated by power devices.
Junction-to-case thermal resistance is specified from
the IC junction to the bottom of the case directly below
the die.
This is the lowest resistance path for heat flow.
Proper mounting is required to ensure the best possible
thermal flow from this area of the package to the heat
sinking material. Note that the Exposed Pad is electrically
connected to the output.
Figure 7. Connections for Best Load Regulation
+
–
LT3080-1
IN
V
CONTROL
OUT
30801 F07
SET
R
SET
R
P
25mΩ
PARASITIC
RESISTANCE
R
P
R
P
LOAD
Load Regulation
Because the LT3080-1 is a floating device (there is no
ground pin on the part, all quiescent and drive current is
delivered to the load), it is not possible to provide true
remote load sensing. Load regulation will be limited by the
resistance of the connections between the regulator and
the load. The data sheet specification for load regulation
is Kelvin sensed at the pins of the package. Negative side
sensing is a true Kelvin connection, with the bottom of
the voltage setting resistor returned to the negative side of
the load (see Figure 7). Connected as shown, system load
regulation will be the sum of the LT3080-1 load regulation
and the parasitic line resistance multiplied by the output
current. It is important to keep the positive connection
between the regulator and load as short as possible and
use large wire or PC board traces.
applicaTions inFormaTion
The internal 25mΩ ballast resistor is outside of the
LT3080-1’s feedback loop. Therefore, the voltage drop
across the ballast resistor appears as additional DC load
regulation.
However, this additional load regulation can
actually improve transient response performance by de
-
creasing peak-to-peak output voltage deviation and even
save on total output capacitance.
This technique is called
active voltage positioning and is especially useful for ap
-
plications that must withstand large output load current
transients. For more information, see Design Note 224,
“Active Voltage Positioning Reduces Output Capacitors.”
The basic principle uses the fact that output voltage is
a function of output load current. Output voltage is set
based on the midpoint of the output load current range:
• I
OUT(MIN)
+I
OUT(MAX)
( )