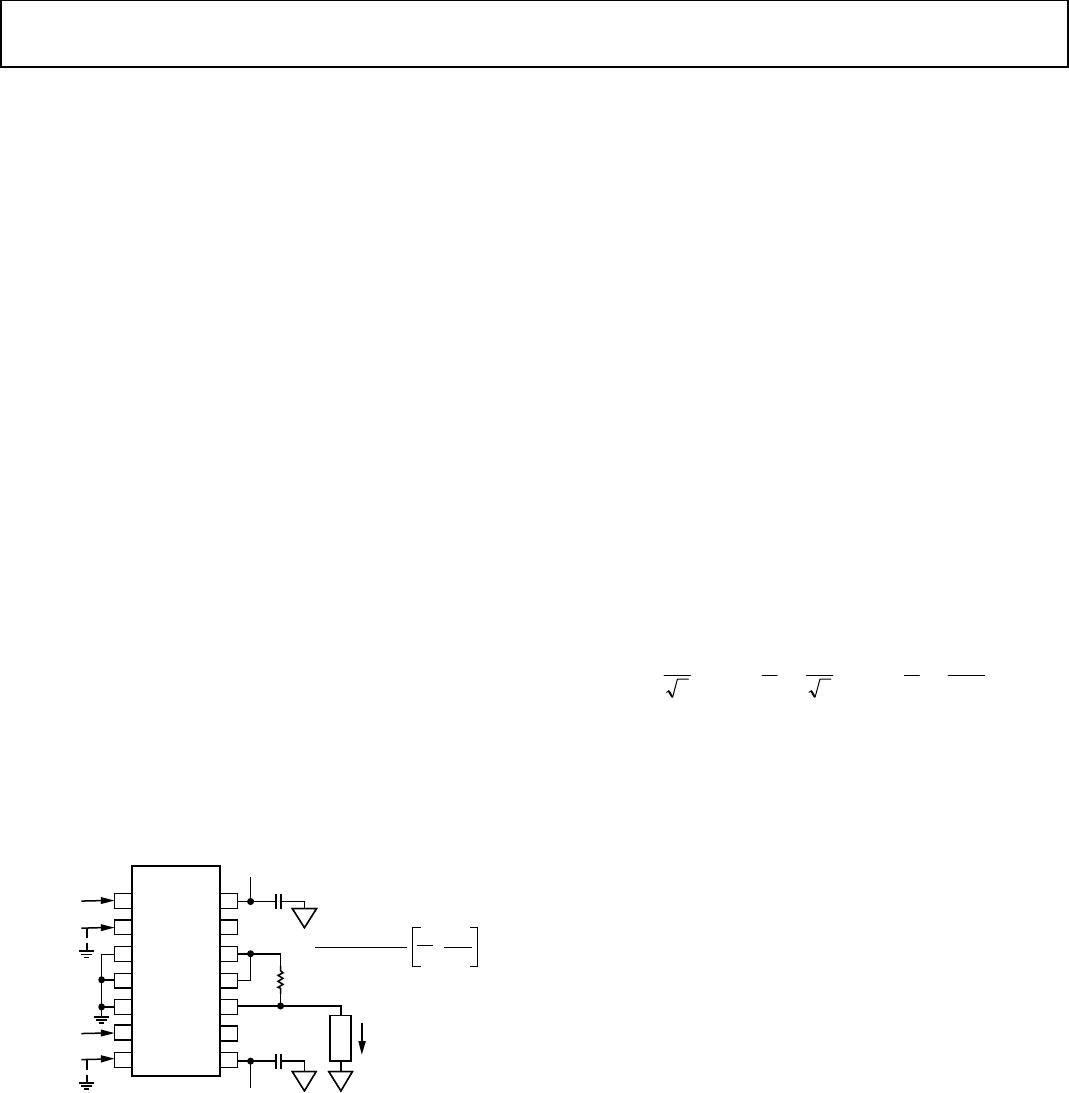
AD734
Rev. E | Page 13 of 20
At least one of the two inputs of any pair must be provided with
a dc path (usually to ground). The careful selection of ground
returns is important in realizing the full accuracy of the AD734.
The Z2 pin is normally connected to the load ground, which can be
remote in some cases. It can also be used as an optional summing
input (see Equation 3 and Equation 4) having a nominal FS
input of ±10 V and the full 10 MHz bandwidth.
In applications where high absolute accuracy is essential, the
scaling error caused by the finite resistance of the signal source(s)
may be troublesome; for example, a 50 Ω source resistance at
just one input introduces a gain error of −0.1%; if both the X
and Y inputs are driven from 50 Ω sources, the scaling error in
the product is −0.2%. If the source resistances are known, this
gain error can be completely compensated by including the
appropriate resistance (50 Ω or 100 Ω, respectively, in the
preceding cases) between the output, W (Pin 12), and the Z1
feedback input (Pin 11). If Rx is the total source resistance
associated with the X1 and X2 inputs, and Ry is the total source
resistance associated with the Y1 and Y2 inputs, and neither Rx
nor Ry exceeds 1 kΩ, a resistance of Rx + Ry in series with
Pin Z1 provides the required gain restoration.
Pin 9 (ER) and Pin 13 (DD) should be left unconnected in this
application. The U inputs (Pin 3, Pin 4, and Pin 5) are shown
connected to ground; they can alternatively be connected to
VN, if desired. In applications where Pin 2 (X2) happens to
be driven with a high amplitude, high frequency signal, the
capacitive coupling to the denominator control circuitry via
an ungrounded Pin 3 can cause high frequency distortion.
However, the AD734 can be operated without modification in
an
AD534 socket and these three pins left unconnected with the
preceding caution noted.
AD734
X1
1
X2
2
U0
3
U1
4
U2
5
VP
14
DD
NC
NC
13
W
12
Z1
11
Z2
10
Y2
7
ER
9
VN
8
Y1
6
L
L
X INPUT
±10V FS
Y INPUT
±10V FS
+15
R
S
–15V
0.1µF
0.1µF
L
LOAD
±10mA MAX FS
±10V MAXIMUM
LOAD VOLTAGE
I
W
I
W
=
(X
1
– X
2
)(Y
1
–Y
2
)
10V
+
1
R
S
1
50kΩ
0827-008
Figure 25. Conversion of Output to a Current
Current Output
It may occasionally be desirable to convert the output voltage to
a current. In correlation applications, for example, multiplication is
followed by integration; if the output is in the form of a current,
a simple grounded capacitor can perform this function. Figure 25
shows how this can be achieved. The op amp forces the voltage
across Z1 and Z2, and thus across the resistor, RS, to be the
product XY/U. Note that the input resistance of the Z interface
is in shunt with RS, which must be calculated accordingly.
The smallest FS current is simply ±10 V/50 kΩ, or ±200 μA,
with a tolerance of about 20%. To guarantee a 1% conversion
tolerance without adjustment, R
S
must be less than 2.5 kΩ. The
maximum full-scale output current should be limited to about
±10 mA (thus, R
S
= 1 kΩ). This concept can be applied to all
connection modes, with the appropriate choice of terminals.
Squaring and Frequency-Doubling
Squaring of an input signal, E, is achieved by connecting the X
and Y inputs in parallel; the phasing can be chosen to produce
an output of E
2
/U or −E
2
/U as desired. The input can have
either polarity, but the basic output is either always positive or
negative; as for multiplication, the Z2 input can be used to add a
further signal to the output.
When the input is a sine wave, a squarer behaves as a frequency
doubler, because
(Esinwt)
2
= E
2
(1 − cos2wt)/2 (8)
Equation 8 shows a dc term at the output, which varies strongly
with the amplitude of the input, E. This dc term can be avoided
using the connection shown in Figure 26, where an RC network
is used to generate two signals whose product has no dc term.
The output is
⎟
⎟
⎠
⎞
⎜
⎜
⎝
⎛
⎭
⎬
⎫
⎩
⎨
⎧
⎟
⎠
⎞
⎜
⎝
⎛
−
⎭
⎬
⎫
⎩
⎨
⎧
⎟
⎠
⎞
⎜
⎝
⎛
+=
V10
1
4
sin
2
4
sin
2
4
ππ
wt
E
wt
E
W
(9)
for w = 1/CR1, which is just
W = E
2
(cos2wt)/(10 V) (10)
which has no dc component. To restore the output to ±10 V
when E = 10 V, a feedback attenuator with an approximate ratio
of 4 is used between W and Z1; this technique can be used
wherever it is desired to achieve a higher overall gain in the
transfer function.
The values of R3 and R4 include additional compensation for the
effects of the 50 kΩ input resistance of all three interfaces; R2 is
included for a similar reason. These resistor values should not
be altered without careful calculation of the consequences. With
the values shown, the center frequency f
0
is 100 kHz for C =
1 nF. The amplitude of the output is only a weak function of
frequency; the output amplitude is 0.5% too low at f = 0.9f
0
and
f = 1.1f
0
. The cross-connection is simply to produce the cosine
output with the sign shown in Equation 10; however, the sign in
this case is rarely important.